16:00 |
0458. |
Structure Tensor Analysis
of Histological Images to Examine Brain Microstructure 
Matthew D Budde1
1Neurosurgery, Medical College of Wisconsin,
Milwaukee, Wisconsin, United States
Accurate methods of validating diffusion MRI findings
are notoriously difficult and lacking. We performed
structure tensor (ST) analysis of digital histological
images to visualize and quantify brain microstructure on
different scales and compare the results to those
obtained from DTI. In the normal rat brain, anisotropy
derived from the disparate modalities was highly
correlated and ST analysis permitted putative crossing
fibers to identified and quantified. The method has the
potential to accurately validate diffusion MRI findings
in the normal and injured or diseased central nervous
system.
|
16:12 |
0459. |
Exploiting non-Gaussian
phase distributions to model micron-scale restricted
diffusion 
Leigh A. Johnston1,2, David Wright2,3,
and Iven M. Mareels1
1NeuroEngineering Laboratory, Electrical and
Electronic Engineering, University of Melbourne,
Parkville, VIC, Australia, 2Florey
Neuroscience Institutes, Parkville, VIC, Australia, 3Centre
for Neuroscience, University of Melbourne, Parkville,
VIC, Australia
A model of cylindrically restricted diffusion is derived
through analytic combination of non-Gaussian phase
distribution, applicable to the micron-size axon
compartments and diffusion characteristics of mammalian
white matter, with an assumed parametric form for the
cylinder radii probability density function. The
resultant model is presented as an alternative to the
AxCaliber method of Assaf et al (2008) that obviates the
need for truncation of Bessel functions to solve. We
apply the restricted diffusion form in a two-compartment
model to two experimental datasets, rat auditory nerve
and sheep brain, and demonstrate its ability to infer
axon diameter densities.
|
16:24 |
0460.
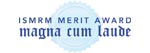 |
Diffusion enables the
distinction between types of tissue microstructure by
analyzing the water lineshape 
Alexander Ruh1, Philipp Emerich1,
Dmitry S. Novikov2, and Valerij G. Kiselev1
1Department of Radiology, Medical Physics,
University Medical Center Freiburg, Freiburg, Germany, 2Center
for Biomedical Imaging, Department of Radiology, NYU
School of Medicine, New York, NY, United States
We show analytically and numerically, that the NMR
spectral lineshape can distinguish between different
kinds of global structural organization (order and
various types of disorder) of tissue susceptibility.
This distinction involves long-range correlations and is
thereby robust to the biological variability. The
biophysical mechanism behind this phenomenon is the
molecular diffusion mediating the spin dephasing
reflected in the transverse relaxation. Our results
provide a framework for novel types of
susceptibility-based contrast.
|
16:36 |
0461.
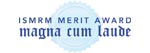 |
Imaging the pore density
function by synergistic diffusion-diffractions 
Noam Shemesh1, and Yoram Cohen1
1School of Chemistry, Tel Aviv University,
Tel Aviv, Israel
Diffusion-diffractions are unique reporters for
compartment morphology in porous systems. In
single-Pulsed-Field-Gradient (s-PFG) MR the signal decay
E(q), is in fact the FT of the averaged propagator,
which reports on the compartment size. By contrast, the
pore density function, ñ(r), holds all the information
on the compartment morphology including size and shape;
however, in conventional s-PFG MR, this quantity is
intractable. Here, we show that by synergistic
application of the diffraction patterns of double-PFG
and s-PFG MR, one can in fact obtain the pore density
function. Thus, an image of the pore space is obtained,
without performing traditional MR imaging.
|
16:48 |
0462. |
In-vivo angular double-PFG
MRI of the human brain 
Carl-Fredrik Westin1, Markus Nilsson2,
Ofer Pasternak1, Daniel Topgaard3,
and Hans Knutsson4
1Department of Radiology, BWH, Harvard
Medical School, Boston, MA, United States, 2Department
of Medical Radiation Physics, Lund University, Lund,
Sweden, 3Division
of Physical Chemistry, Lund University, Lund, Sweden, 4Department
of Biomedical Engineering, Medical Informatics,
Linköping University, Linköping, Sweden
Previous experimental results demonstrate that angular
double-PFG analysis alleviates the demand for strong
gradients for microstructure determination and that
estimation of novel features of tissue that displays a
microscopic anisotropy may be possible using clinical
scanners. We here present new data supporting this
claim. The presented work shows that it is possible to
perform in vivo double PFG imaging of the human brain
with a good SNR, indicating that the new the
microstructural contrasts from double-PFG can be made
available to studies of clinical populations.
|
17:00 |
0463. |
NODDI: a practical
technique for in vivo neurite orientation dispersion and
density imaging of the human brain 
Hui Zhang1, Torben Schneider2,
Claudia AM Wheeler-Kingshott2, and Daniel C
Alexander1
1Department of Computer Science & Centre for
Medical Image Computing, University College London,
London, United Kingdom, 2NMR
Research Unit, Department of Neuroinflammation, UCL
Institute of Neurology, University College London,
London, United Kingdom
This work proposes neurite orientation dispersion and
density imaging (NODDI), a practical diffusion MRI
technique for quantifying microstructural complexity of
dendrites and axons on clinical scanners. Orientation
dispersion and density are two key microstructural
features of neurites. Ex vivo studies have shown that
estimates of these features from diffusion MRI are
consistent with independent histological measures.
However, existing protocols are impractical for clinical
applications. We address this problem by developing
NODDI, a novel neurite imaging and analysis framework
with clinical feasibility. We demonstrate that NODDI
enables for the first time the whole-brain in vivo
imaging of neurite characteristics.
|
17:12 |
0464.
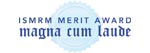 |
Detection of Microscopic
Diffusion Anisotropy in the Living Human Brain 
Marco Lawrenz1, and Jürgen Finsterbusch1
1Institute of Systems Neuroscience,
University Medical Center Hamburg-Eppendorf, Hamburg,
Germany, Germany
Anisotropy measures derived from DTI reflect a mixture
of cell eccentricity and their orientation distribution
within the voxel which hampers their informative value.
For instance, a vanishing FA in brain white matter is
consistent with the absence of eccentric cells like
neurons but also with a uniform orientation distribution
of neuronal fibers. Here, it is shown that
double-wave-vector diffusion-weighting experiments can
detect the diffusion anisotropy on a microscopic level
in the living human brain. In particular, it is
demonstrated that the microscopic anisotropy can also be
observed in regions-of-interest that are macroscopically
isotropic, i.e. with a FA value of 0.
|
17:24 |
0465.
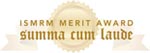 |
Diffusion distinguishes
between axonal loss and demyelination in brain white matter 
Els Fieremans1, Jens H. Jensen2,
Joseph A. Helpern2, Sungheon Kim1,
Robert I. Grossman1, Matilde Inglese3,
and Dmitry S. Novikov1
1Center for Biomedical Imaging, Radiology,
New York University School of Medicine, New York, NY,
United States, 2Radiology
and Radiological Science, Medical University of South
Carolina, Charleston, SC, United States, 3Neurology,
Mount Sinai School of Medicine, New York, NY, United
States
Non-Gaussian diffusion MRI methods provide estimates for
the axonal water fraction (AWF) and tortuosity of the
extra-axonal space. Using Monte Carlo simulations in a
realistic geometry of the corpus callosum, we show that
the AWF is most sensitive to axonal loss, whereas the
tortuosity is most sensitive to demyelination. The value
of this distinction is demonstrated by quantifying the
degree of demyelination and axonal loss in multiple
sclerosis and in Alzheimer’s disease (AD), where our
analysis demonstrates that the change from normal to
mild cognitive impairment is mainly explained by
demyelination, while conversion into AD is characterized
by axonal loss.
|
17:36 |
0466.
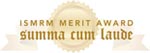 |
Quantifying Axonal Injury,
Demylination, and inflammation in Human MS Autopsy Specimens
using Diffusion Basis Spectrum Imaging (DBSI) 
Yong Wang1, Qing Wang1, Mingqiang
Xie2, Anne H. Cross2, and
Sheng-Kwei Song1
1Radiology, Washington University in St.
Louis, Saint Louis, MO, United States, 2Neurology,
Washington University in St. Louis, Saint Louis, MO,
United States
During CNS pathology, cellularity is enhanced due to
increased numbers of microglia, astrocytes and
infiltrating inflammatory cells such as macrophages and
lymphocytes. Presence of these cells could potentially
confound white matter pathology as assessed using DTI.
Diffusion basis spectrum imaging (DBSI) has been
developed to accurately quantify the extent of
cellularity as well as the degree of axonal injury and
demyelination in a cuprizone-treated mouse model. In
this study, autopsy spinal cord specimens of MS patients
were examined to correlate DBSI findings with
immunohistochemistry (IHC). Preliminary data indicated
that DBSI findings are consistent with IHC results,
supporting its clinical application.
|
17:48 |
0467. |
Diffusion MRI measurement
of Axon Diameter alterations induced by White Matter
Plasticity 
Lea Vinokur1, Daniel Barazany1,
Shimrit Tsur-Moryosef1, and Yaniv Assaf1
1Neurobiology, Tel Aviv University, Tel Aviv,
Israel
In this study our goal was to measure structural
plasticity related changes in white matter induced by
spatial learning. Our focus was on the Corpus Callosum
of rats that underwent a spatial learning and memory
test. We used AxCaliber, an advanced diffusion MRI
method to extract morphological parameters of the
tissue, such as the Axon Diamter Distribution (ADD) and
axonal density. Based on the retrieved parameters the
CC's are clustered into 6 clusters using the k-means
clustering algorithm. Statistical analysis was performed
on each cluster, comparing the learning group to two
control groups.
|
|