Wednesday Parallel 4 Live Q&A |
Wednesday, 12 August 2020, 15:15 - 16:00 UTC |
Moderators: Jennifer McNab |
Session Number: O-69
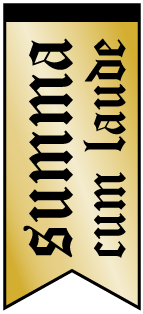 |
0953.
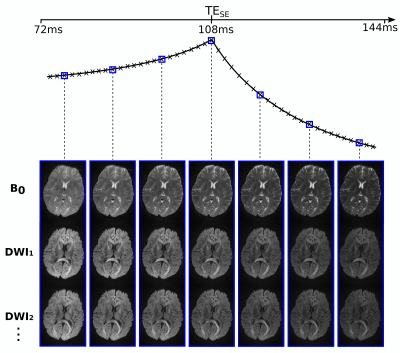 |
Diffusion-PEPTIDE: rapid distortion-free diffusion-relaxometry imaging
Merlin J Fair1,2, Congyu Liao1,2, Daeun Kim3, Divya Varadarajan1,2, Justin P Haldar3,4, and Kawin Setsompop1,2,5
1A. A. Martinos Center for Biomedical Imaging, Massachusetts General Hospital, Charlestown, MA, United States, 2Department of Radiology, Harvard Medical School, Boston, MA, United States, 3Department of Electrical and Computer Engineering, University of Southern California, Los Angeles, CA, United States, 4Department of Biomedical Engineering, University of Southern California, Los Angeles, CA, United States, 5Harvard-MIT Health Sciences and Technology, MIT, Cambridge, MA, United States
Watch the Video
Diffusion-PEPTIDE incorporates the recently developed rapid multi-shot relaxometry technique Propeller EPTI with Dynamic Encoding (PEPTIDE) into a diffusion acquisition scheme. PEPTIDE enables fast acquisition of distortion- and blurring-free images, time-resolved for different timepoints with varying T2 & T2* weighting, with self-navigation for correction of shot-to-shot phase-variation and motion. Diffusion-PEPTIDE is demonstrated here to enable distortion-free in vivo diffusion-relaxometry with large parameter space in an sensible acquisition time.
|
|
0954.
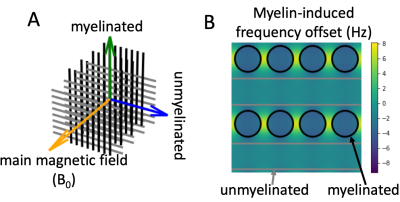 |
Diffusion-weighted phase imaging: towards a tract-specific myelin measure
Michiel Cottaar1, Benjamin C. Tendler1, Wenchuan Wu1, Karla L. Miller1, and Saad Jbabdi1
1WIN@FMRIB, University of Oxford, Oxford, United Kingdom
Watch the Video
We propose a novel sequence that adds a second asymmetric spin echo after a standard Stejskal-Tanner sequence. This allows the estimation of the off-resonance frequency of the diffusion-weighted signal due to the myelin magnetic susceptibility. Varying the orientation of the diffusion-weighting gradient dephases different fibre populations. In simulations we show that for a sufficiently high b-value (>~3 ms/μm2), the intra-axonal water will dominate leading to a simple relation between the myelin-induced frequency shift and the log g-ratio. This allows the difference in log g-ratio between crossing fibres to be measured and hence estimate the myelination of individual crossing tracts.
|
|
0955.
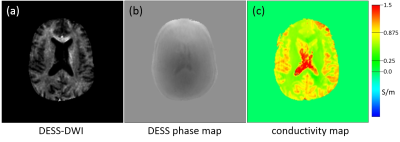 |
Simultaneous acquisition of diffusion weighted images and conductivity maps using a balanced double echo steady state (DESS) sequence
Jochen Keupp1, Bernhard Gleich1, and Ulrich Katscher1
1Philips Research, Hamburg, Germany
Watch the Video
A combined acquisition of distortion-free diffusion-weighted images and tissue conductivity maps is explored using a fully balanced double echo steady state (DESS) sequence. Banding artifacts are avoided using sufficiently high gradient moments of the diffusion gradient, such that the banding is contained within single voxels. The stability of the B1 transceive phase measurement by the balanced DESS sequence allows the derivation of quantitative tissue conductivity based second derivative using standard EPT (electrical properties tomography) methods. Feasibility of simultaneous DWI and EPT is shown on a 3T MRI system in phantom and volunteer experiments (head).
|
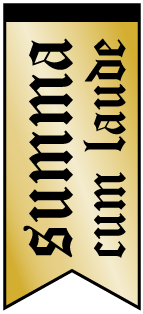 |
0956.
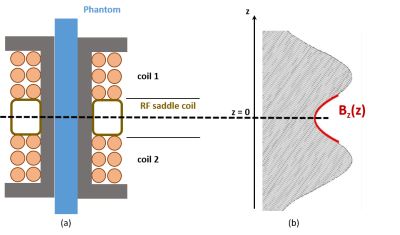 |
Diffusion phase-imaging using non-linear gradients in anisotropic synthetic fiber phantoms
Pamela Wochner1, Torben Schneider2, Jason Stockmann3, Jack Lee1, and Ralph Sinkus1,4
1School of Biomedical Engineering & Imaging Sciences, King's College London, London, United Kingdom, 2Philips Healthare, Guildford, United Kingdom, 3Department of Radiology, Massachusetts General Hospital, Athinoula A. Martinos Center for Biomedical Imaging, Charlestown, MA, United States, 4Inserm U1148, LVTS, University Paris Diderot, Paris, France
Watch the Video
Diffusion MRI classically uses linear gradients to encode information about micro-structure in the loss of signal magnitude. When replaced by gradients varying quadratically in space, anisotropic diffusion results in a net phase shift, while the signal magnitude is largely preserved. This allows the extraction of information from signal phase inaccessible to other diffusion MRI methods. The phase evolution of anisotropic fiber phantoms were studied in simulations and diffusion experiments. Simulations confirm increasing phase change with increasing anisotropy and mixing time between diffusion gradients. First MR experiments with different mixing times show a phase shift in good agreement with theoretical estimate.
|
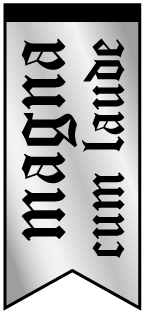 |
0957.
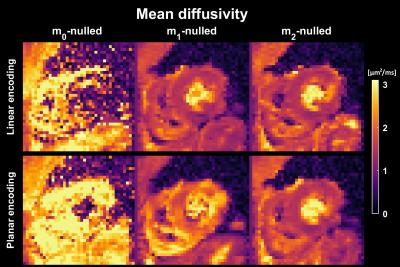 |
Motion-compensated gradient waveform design for tensor-valued diffusion encoding by constrained numerical optimization
Filip Szczepankiewicz1,2,3, Irvin Teh4, Erica Dall'Armellina4, Sven Plein4, Jurgen E. Schneider4, and Carl-Fredrik Westin2,3
1Clinical Sciences Lund, Lund University, Lund, Sweden, 2Radiology, Brigham and Women's Hospital, Boston, MA, United States, 3Harvard Medical School, Boston, MA, United States, 4Leeds Institute of Cardiovascular and Metabolic Medicine, University of Leeds, Leeds, United Kingdom
Watch the Video
Motion compensation is vital for cardiac diffusion MRI. In this paper we propose an optimized gradient waveform design that allows tensor-valued diffusion encoding with motion compensation. We demonstrate that it works for in vivo cardiac imaging and we show that it is more efficient than previous designs.
|
|
0958.
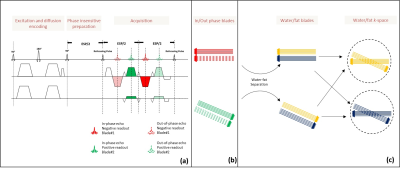 |
A TSE BLADE based distortion-free diffusion-weighted imaging method with Dixon water-fat separation
Kun Zhou1, Wei Liu1, and Yulin V Chang2
1Siemens Shenzhen Magnetic Resonance Ltd., Shenzhen, China, 2Siemens Medical Solutions USA, Boston, MA, United States
Watch the Video
Diffusion weighted imaging with EPI can suffer from image distortions due to sensitivity to B0 inhomogeneity and chemical shift related artifacts induced by incomplete fat suppression. In this study, we propose a TSE BLADE sequence with Dixon water-fat separation for DWI. With this technique, distortion-free DWI with robust fat suppression was shown to be feasible, even in body regions with strong B0 inhomogeneity.
|
|
0959.
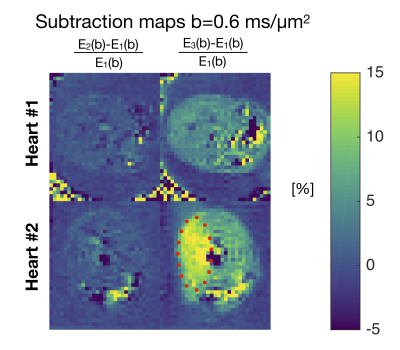 |
Stay on the beat: tuning in on time-dependent diffusion in the heart
Henrik Lundell1, Samo Lasič1,2, Filip Szczepankiewicz3,4,5, Markus Nilsson3, Daniel Topgaard6, Jürgen E. Schneider7, and Irvin Teh7
1Danish Research Centre for Magnetic Resonance, Centre for Functional and Diagnostic Imaging and Research, Copenhagen University Hospital Hvidovre, Hvidovre, Denmark, 2Random Walk Imaging AB, Lund, Denmark, 3Clinical Sciences, Lund University, Lund, Sweden, 4Harvard Medical School, Boston, MA, United States, 5Brigham and Women's Hospital, Boston, MA, United States, 6Physical Chemistry, Lund University, Lund, Sweden, 7Leeds Institute of Cardiovascular and Metabolic Medicine, University of Leeds, Leeds, United Kingdom
Watch the Video
Diffusion encoding with general gradient waveforms provides flexibility and experimental efficiency for multidimensional diffusion encoding (MDE). Here we investigate b-tensor shape and spectral content as two independent measurement dimensions for imaging myocardial microstructure. By tuning spectral content, we demonstrate that time-dependent diffusion can be controlled for across b-tensor shapes and that tuning in itself provide a strong image contrast in a clinically feasible setting. For encoding high frequencies alone, our isotropic encoding provides higher experimental efficiency.
|
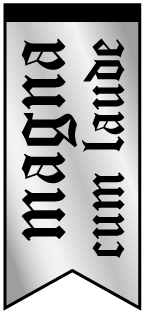 |
0960.
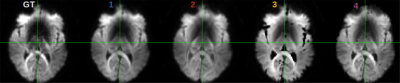 |
Acquiring and predicting MUlti-dimensional DIffusion (MUDI) data: an open challenge
Marco Pizzolato1, Marco Palombo2, Elisenda Bonet-Carne2,3, Francesco Grussu2,4, Andrada Ianus5, Fabian Bogusz6, Tomasz Pieciak6,7, Lipeng Ning8, Stefano B. Blumberg2, Thomy Mertzanidou2, Daniel C. Alexander2, Maryam Afzali9, Santiago Aja-Fernández7,9,
Derek K. Jones9,10, Carl-Fredrik Westin8, Yogesh Rathi8, Steven H. Baete11,12, Lucilio Cordero-Grande13, Thilo Ladner14, Paddy J. Slator2, Daan Christiaens13,15, Jean-Philippe Thiran1,16, Anthony N. Price13, Farshid Sepehrband17, Fan Zhang8, and Jana Hutter13
1Signal Processing Lab (LTS5), École Polytechnique Fédérale de Lausanne, Lausanne, Switzerland, 2Department of Computer Science, Centre for Medical Image Computing, University College London, London, United Kingdom, 3BCNatal Fetal Medicine Research Center, Barcelona, Spain, 4Queen Square MS Centre, UCL Queen Square Institute of Neurology, Faculty of Brain Sciences, University College London, London, United Kingdom, 5Champalimaud Research, Champalimaud Centre for the Unknown, Lisbon, Portugal, 6AGH University of Science and Technology, Kraków, Poland, 7Laboratorio de Procesado de Imagen (LPI), Universidad de Valladolid, Valladolid, Spain, 8Brigham and Women’s Hospital, Harvard Medical School, Boston, MA, United States, 9Cardiff University Brain
Research Imaging Center (CUBRIC), School of Psychology, University of Cardiff, Cardiff, United Kingdom, 10Mary MacKillop Institute for Health Research, Faculty of Health Sciences, Australian Catholic University, Melbourne, Australia, 11Center for Biomedical Imaging, Dept. of Radiology, New York University School of Medicine, New York, NY, United States, 12Center for Advanced Imaging Imaging, Innovation and Research, New York University School of Medicine, New York, NY, United States, 13Centre for Medical Engineering, Centre for the Developing Brain, King's College London, London, United Kingdom, 14Department of Chemistry and Applied Biosciences, ETH Zurich, Zurich, Switzerland, 15Department of Electrical Engineering (ESAT-PSI), KU Leuven, Leuven, Belgium, 16Radiology Department, Centre Hospitalier Universitaire Vaudois and University of Lausanne, Lausanne, Switzerland, 17Laboratory
of Neuro Imaging (LONI), USC Stevens Neuroimaging and Informatics Institute, University of Southern California, Los Angeles, CA, United States
Watch the Video
The variety of possible combinations of acquisition parameters is key to the versatility of MRI as a diagnostic modality. However, the full exploration of the parameter space defined by b-values, gradient directions, inversion and echo times comes at the expense of the acquisition time. We present the results of an open challenge where different methods were proposed to predict the content of a densely sampled acquisition, which explores such parameter space, from only a subset of parameter combinations. These indicate the possibility of leveraging the redundancy in the data to shorten the acquisition time while minimizing information loss.
|
|
0961.
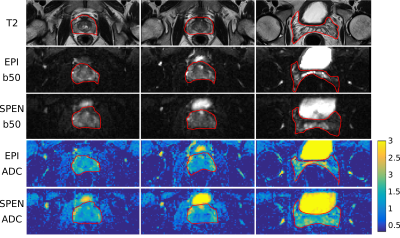 |
Overcoming geometric distortions in human prostate diffusion weighted imaging by spatio-temporal encoded (SPEN) MRI
Martins Otikovs1, Lingceng Ma1, and Lucio Frydman1
1Weizmann Institute of Science, Rehovot, Israel
Watch the Video
Spatiotemporal encoding (SPEN) is an alternative ultrafast imaging technique which allows one to manipulate the bandwidth along the phase-encoding (PE) direction as well as to achieve T2* refocusing throughout the FID acquisition, thereby overcoming distortions observed along EPI’s PE dimension. The study compares multislice 2D SPEN and a 3D SPEN sequence variants against EPI derivatives, evaluating their ability to deliver prostate diffusion-weighted imaging (DWI) data and apparent diffusion coefficient (ADC) maps on healthy human volunteers. Essentially distortion-free diffusion weighted images and ADC maps of prostate with good SNR were achieved by the 2D SPEN variant.
|
|
0962.
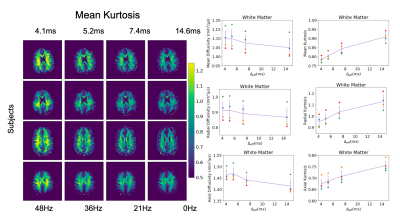 |
Measuring Time-Dependent Diffusion Kurtosis Using the MAGNUS High-Performance Head Gradient
Grant Kaijuin Yang1,2, Ek Tsoon Tan3, Eric Fiveland4, Thomas Foo4, and Jennifer McNab2
1Electrical Engineering, Stanford University, Stanford, CA, United States, 2Radiology, Stanford University, Stanford, CA, United States, 3Hospital for Special Surgery in Manhattan, New York, NY, United States, 4GE Global Research, Niskayuna, NY, United States
Watch the Video
In this work, we measure time-dependent effects on diffusion kurtosis estimates in the in vivo human brain over an extended range of b-values(458-2000s/mm2) and frequencies(0-96Hz) using a high-performance head gradient coil on a whole-body 3T MRI.
|
|